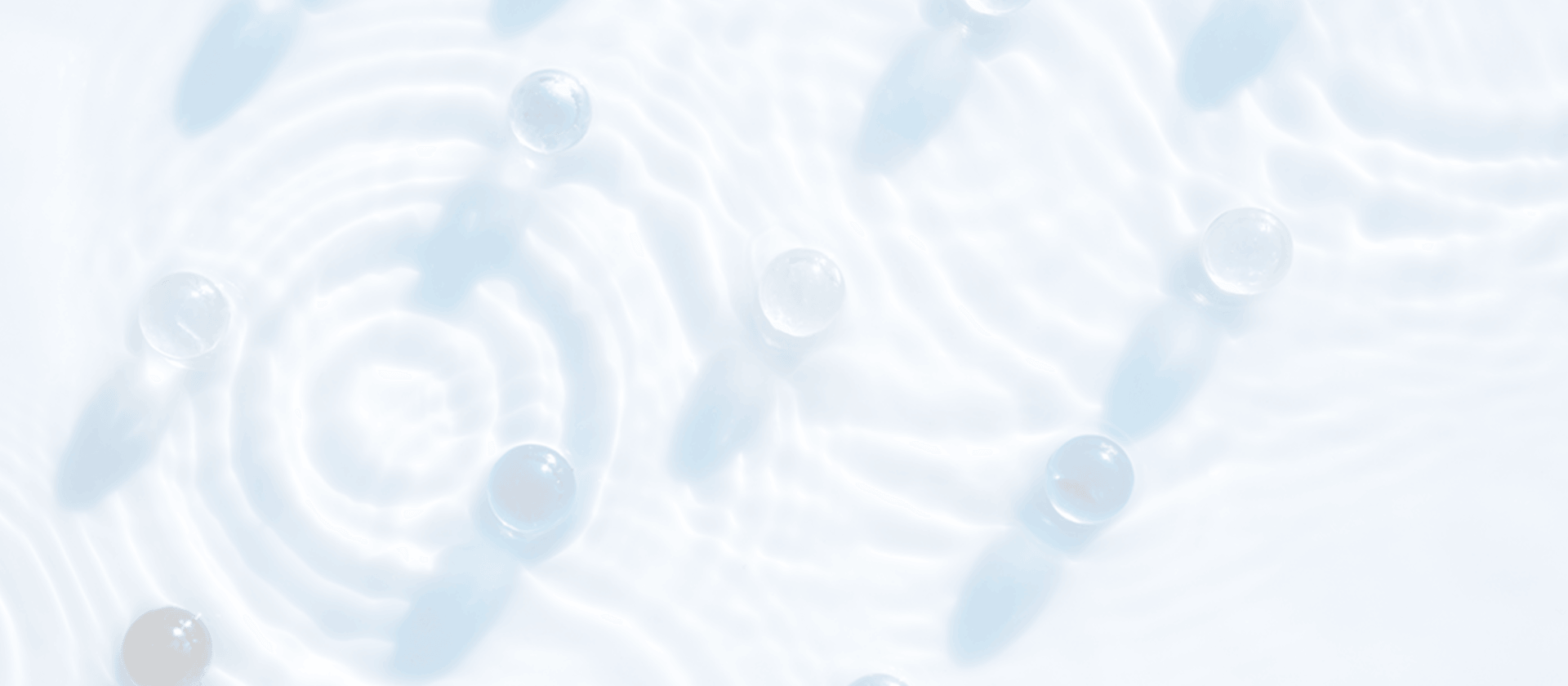
HISTORY
Bioactive glasses are one of the synthetic biomaterials that can bind to bone completely. It was developed in the late 1960s by Larry L. Hench. Dr. While working at the University of Florida in Hench, Colonel Klinker, who was working in the United States on a bus journey to attend the US Army Materials Research Conference held in Sagamore, New York, said, ‘If you can make a material that can withstand exposure to high-energy radiation Hench realized the need for a new material that can create a living bond with the tissues in the body. When Hench returned to Florida after the conference, he presented a product proposal to the US Army Medical Research and Design Command, based on body theory, rejecting metallic or polymeric material unless it could form a hydroxyapatite coating found in bone. In 1968, he received funding from the US Army Medical Research and Design Command, and in November 1969 Hench began synthesizing small rectangles of what he called 45S5 bioactive glass. The product was then first implanted into rat femurs at the VA Hospital in Gainesville. Six weeks later, hospital officer Greenlee called Hench, ‘‘ Larry, what are the examples you gave me? They will not come out of bone. “I pulled them, pushed them, broke the bone, and it’s still tied in place.” Bioactive glass was born with this first successful experiment and the first compositions were studied. Hench published his first paper on the subject in the Journal of Biomedical Materials Research in 1971, and his laboratory continued to work on the project for the next 10 years with continued funding from the US Army. Until 2006, over 500 articles on bioactive glasses were published from different laboratories and institutions around the world. The first successful surgical use of bioactive glass 45S5 was to replace the ossicles in the middle ear as a treatment for conductive hearing loss, and the material continues to be used in bone reconstruction applications today.
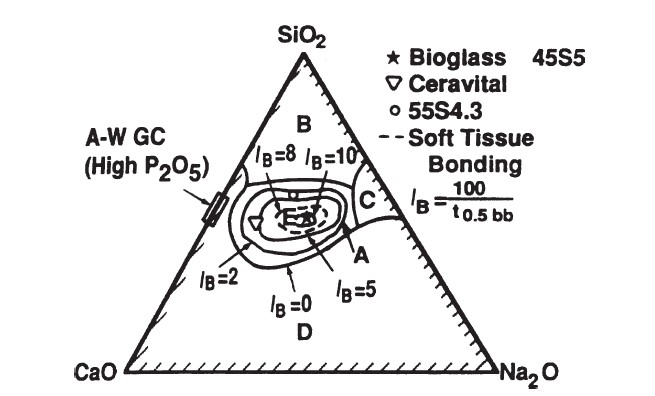
45S5 PHASE DIAGRAM
The compositional dependence (in% by weight) of bone bonding and soft tissue bonding of bioactive glasses and glass ceramics is included in the above phase diagram. All compositions in zone A have a fixed weight of 6% P2O5. BONE-G ACTIVE® has the properties in the region indicated by ★ in the phase diagram above.
★ |
⇒ |
⇒ |
SiO2 : 45 % | |
BONE-G ACTIVE® | Na2O : 24.5 % | |||
Bioactive glass 45S5 | CaO : 24.5 % | |||
P2O5: 6 % |
Bioactive glasses, which are the first biomaterial group known for their bone bonding and most commonly known as 45S5, contain certain proportions of SiO2, Na2O, CaO and P2O5 composition. The 45S5 bioactive glass formulation expresses 45% SiO2 by weight and a 5: 1 CaO / P2O5 ratio. There are three basic compositional properties that distinguish bioactive glasses from conventional soda-lime-silica glasses. These are less than 60 mol% SiO2, high Na2O and CaO content, and a high CaO / P2O5 ratio. Thanks to these properties, the bioactive glass surface becomes highly reactive when exposed to an aqueous environment. Glasses with lower CaO – P2O5 ratios cannot stick to bone. In the bone bonding process, the bioactive glass surface forms a biologically active carbonated HA layer (HCA) that provides the bonding interface with tissues. In this way, it develops a sticky interface with tissues that resists mechanical forces. The compositional dependence of bone and soft tissue bond on Na2O – CaO – P2O5 – SiO2 glasses is shown in the phase diagram in the figure. All glasses in the diagram contain a fixed ratio of 6% P2O5 by weight. The compounds in the A region indicated in the diagram are bioactive glasses that exhibit a level of bioactivity capable of forming a strong bond with bone. For this reason, zone A is called the bioactive bone attachment border. The B region is defined as the region of bioinert glasses that do not bind to bone. However, silicate glasses in the B zone behave like inert materials and reveal a fibrous capsule at the implant-tissue interface. Glasses in the C region are resorbable and disappear within 10 to 30 days after implantation. Glasses in zone D are technically impractical. Therefore it has not been tested as implants. In addition, the collagen component of soft tissues can adhere strongly to bioactive silicate glasses located within the dotted line region shown in the figure.
IMPACT MECHANISM
When bioactive glass 45S5 is implanted, it reacts with the surrounding physiological fluid and causes the formation of a hydroxyl carbonate apatite (HCA) layer on the material surface. The HCA layer has a composition similar to hydroxyapatite, the mineral phase of bone. It has a quality that allows strong interaction and integration with the bone. This reaction is related to the bioactive glass reaction in the environment within the body and takes place rapidly on the material surface within a few hours. The reaction steps take place as follows,
- Calcium and phosphate ions in bioactive glass rapidly change location with body fluids from the surface. As a result of this reaction, hydrolysis process takes place in the silica groups containing bioactive glass and pH and osmotic pressure increase in this region.
- The silica groups that are subjected to hydrolysis and dissolved react to form a gel layer on the bioactive glass surface by gelating.
- After the reaction, a gel layer of CaO⎯P2O5 is formed on the bioactive glass surface. This layer contains OH− and CO32− from body solution and causes crystallization. This layer is called carbonated hydroxyl apatite (HCA). HCA is a structure with the same structural components as natural bone.
- As a result of the first HCA formation, Ca and P ions are taken and growth continues spontaneously. Subsequently, the absorption and desorption of biological factors increase.
- Adsorbed growth factors cause the activation of M2 macrophages. M2 macrophages tend to promote wound healing and initiate the migration of progenitor cells to an injury site. Conversely, when a non-biocompatible material is implanted M1 macrophages are activated and trigger an inflammatory response.
- Mesenchymal stem cells and osteoprogenitor cells triggered by M2 macrophage activation migrate to the bioactive glass surface and bind to the HCA layer.
- Stem cells and osteoprogenitor cells on the HCA surface differentiate into osteogenic cells typically found in bone tissue, particularly osteoblasts.
- Bound and differentiated osteoblasts produce and accumulate extracellular matrix (ECM) components, particularly type I collagen, which is the main protein component of bone.
- Collagen ECM is normally mineralized as occurs in natural bone. Nanoscale hydroxyapatite crystals form a layered structure with collagen deposited on the implant surface.
- Following these reactions, bone growth continues while newly harvested cells continue to function and facilitate tissue growth and repair. The bioactive glass implant continues to deteriorate and be transformed into new ECM material.
GRAPHICS and EXPLANATION
Figure 1 Figure 2
Tissue attachment mechanism is directly related to the type of response at the implant-tissue interface. No material implanted in living tissue is inert because all materials elicit a response from living tissue. There are four types of tissue responses and four different ways of attaching the prosthesis to the skeletal system. A comparison of the relative chemical activity of different bioceramic, glass and glass ceramic types is shown in Figure 1. The part shown in the A region of Figure 1 belongs to the relative reactivity part. Relative reactivity is closely related to the rate at which an interfacial bond of bone and ceramic, glass or glass-ceramic implants is formed. The relative reactivity level of an implant affects the thickness of the interface region or layer between material and tissue.
TYPE I
Type I contains almost inert biomaterials. There is progressive development of inert biomaterials by action of a fibrous capsule in soft and hard tissues. The presence of movement at the biomaterial-tissue interface leads to impairment in the function of the implant, the tissue at the interface, or both. Abrasion particles can accelerate the degradation of the tissue-implant interface. The thickness of the non-stick capsule varies depending on both the material and the size of the relative movement.
TYPE II
Contains type II microporous inert biomaterials. The mechanism behind the use of inert microporous materials; tissue growth into the pores on the surface or into the pores formed along the implant. The increased interface area between the implant and the tissues results in an increased resistance to movement of the device within the tissue. The interface is created by living tissue in the pores. This method of attachment is called “biological fixation”. Can withstand more complex stress situations than “morphologically fixed” type 1 implants. The limitation with type 2 porous implants is that the pores must be larger than 50 to 150 μm for tissue to remain viable and healthy. The large interfacial area required for porosity is due to the need to provide a blood supply to the interwoven connective tissue. Also, if micro motion occurs at the interface of a porous implant and tissue is damaged, the blood supply may be interrupted. As a result, tissue death has occurred and inflammation occurs. Interface stability is destroyed.
TYPEIII
Contains TYPE III bioactive materials. Bioactive materials are intermediate between resorbable and bioinert materials. Bioactive material is a material that elicits a specific biological response at the interface of the material, causing a bonding between tissues and material. All of these materials form an interfacial bond with the adjacent tissue. However, the time dependence of bonding, the strength of the bond, the bonding mechanism, and the thickness of the bonding site differ for various materials. Relatively small changes in the composition of a biomaterial can significantly affect whether it is bioinert, reabsorbable, or bioactive.
TYPE IV
TYPE IV are absorbable biomaterials. Absorbable biomaterials are designed to degrade gradually over a period of time and be replaced by native host tissue. This phenomenon results in a very thin or nonexistent interface thickness. If strength and short-term performance requirements can be met, the optimal biomaterial is the solution because natural tissues can repair and replace themselves throughout life. Therefore, absorbable biomaterials are based on biological repair principles. It is also important that an absorbable biomaterial only consists of metabolically acceptable substances, as large amounts of material can be exchanged. This criterion places important limitations on the compositional design of absorbable biomaterials.